Hey Readers! I am so thankful that you are here! Have you ever wondered what actually causes your skeletal muscles to contract? Well, in this week’s blog post, I will give a relatively brief overview of skeletal muscle contraction. I will explain what a “sarcomere” is and how that structure works to generate force in your muscles via the so-called “sliding filament theory.” If you have a background in biology, exercise science, or a related field, you are probably pretty familiar with what a “sarcomere” is. But, maybe this review will be helpful to you anyways! Whether you are well versed in the sliding filament theory or not, this information is good to know because it is the basis of how movement occurs in your body.

General Overview of Skeletal Muscle Cells
Your body is made up of many different types of cells, including muscle cells. Muscle cells, sometimes referred to as muscle fibers, are long, cylindrical cells, between 10 – 100 micrometers in diameter and up to several centimeters in length. Muscle fibers (aka cells) are grouped into fascicles which, in turn, are grouped together to form a complete muscle. Compared to other cell types in your body (e.g. liver cells, skin cells, etc.), muscle cells are rather large, up to ten times the size of an average body cell. Similar to other cells, muscle cells contain many of the same structures, proteins, and organelles; however, there are some notable differences. The sarcoplasm of a muscle cell (i.e. the fluid found inside a muscle cell; referred to as "cytoplasm" in other cell types) contains a large amount of glycosomes, which are granules of stored glycogen. Glycogen is the storage form of glucose in humans, and it can be used to produce energy when needed, such as during exercise. Muscle cells also contain a large amount of myoglobin, which is a red pigment that stores oxygen. Muscle cells are multinucleated, containing more than once nucleus, whereas most other cells in your body are mononucleated (one nucleus). Muscle fibers also contain many copies of mitochondria (i.e. the organelle responsible for creating most of the energy needed in a cell). But, the most notable difference between muscle cells and other cells in the body is the presence of myofibrils inside the muscle cell.
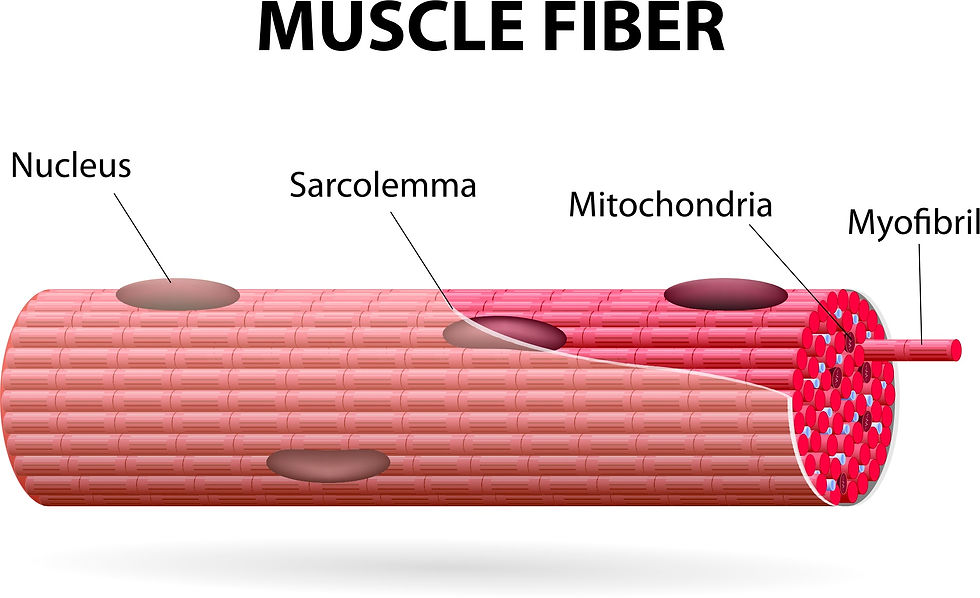
Myofibrils are rodlike structures that run parallel to the length of a skeletal muscle. Hundreds to thousands of myofibrils are packed into a single muscle cell, and they account for about 80% of the volume of a typical muscle cell. The myofibrils contain various proteins organized into repeating sections, where one individual section is known as a sarcomere. Thus, myofibrils are essentially strands of sarcomeres organized in a series. The sarcomere is considered the functional unit and ultimate force generator of the muscle cell. The number of sarcomeres influences the muscle fiber length and cross sectional area (CSA), which, in turn, affects the muscle cell’s force-generating ability.
The Sarcomere - A Deeper Look
Sarocmeres, about 3 micrometers long, are assemblies of proteins and are the contractile units of skeletal muscle. There are three types of proteins within the sarcomere - contractile, regulatory, and structural. The contractile proteins in a sarcomere include actin (a thin stringlike filament) and myosin (a thick filament), and these proteins make up the bulk of the sarcomere. Actin and myosin literally interact to shorten the muscle fiber and generate active force. Structural proteins, such as titin and desmin, provide a supportive infrastructure for the myofibrils and help to transfer forces throughout the muscle. Regulatory proteins, namely tropomyosin and troponin, regulate the interaction between actin and myosin.
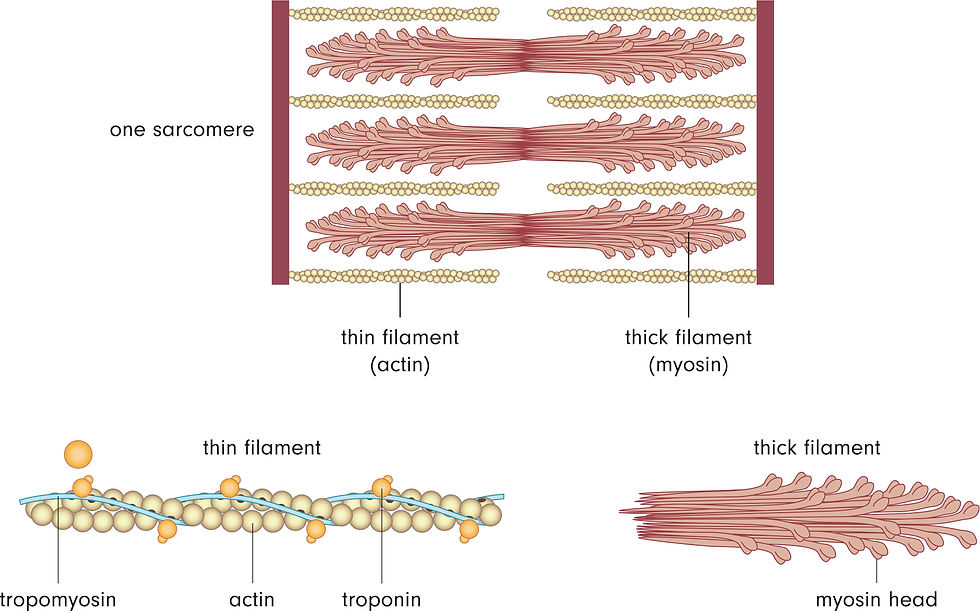
Myosin (i.e. the thick filament in the sarcomere) is composed of a long central part and globular heads, which are projections off the central part. These heads are considered the “business end” of myosin because they link together with actin (i.e. the thin filament in the sarcomere), to create muscle contraction. Actin makes up the bulk of the thin filaments in a sarcomere, and it bears the active site to which the myosin heads attach during contraction.
Tropomyosin is a rod-shaped protein that spirals around the actin. In a relaxed muscle, tropomyosin blocks actin’s active sites so that the myosin heads cannot bind to actin. This is important so that your muscles do not contract when you do not want them to. Conversely, troponin, a small protein, provides a binding site for Calcium, allowing muscle contraction to occur.
The Sliding Filament Theory of Muscle Contraction
This theory states that during muscle contraction, the thin filaments (primarily made of actin) slide past the thick filaments (primarily made of myosin), so that the actin and myosin overlap to a greater degree. Conversely, in a relaxed muscle fiber (or cell), the thick and thin filaments overlap only slightly.
To simplify things greatly, the following actions occur to get a muscle to contract or generate force:
An impulse is sent from the central nervous system (i.e. brain, spinal cord), along a motor neuron, to a muscle.
The impulse is communicated to the muscle cell via the release of Acetylcholine (ACh – a neurotransmitter) across the neuromuscular junction (i.e. the place where a motor nerve meets the muscle).
ACh binds to receptors on the surface of the muscle cell. This ultimately triggers the release of Calcium (Ca2+) inside the muscle cell. Calcium is required for muscles to contract.
The Calcium binds to troponin (one of the regulatory proteins). This causes troponin to change shape, and when this happens, it removes the blocking action of tropomyosin, exposing the binding sites on actin.
Now that the actin binding sites are available and ready for use, the myosin heads can bind and form a cross bridge with the actin. This interdigitation between actin and myosin is the mechanism of force generation in the muscle.
ATP provides the energy to allow the myosin to pull the actin filaments closer together, toward the center of the sarcomere, creating muscle contraction.
The force of this contraction is transmitted to the non-contractile elements surrounding the muscle (i.e. fascia, a type of connective tissue). The fascia then transmits the force to the tendon (which attaches muscle to bone), and then on to the bone, moving the joint.
Once intracellular Calcium levels drop low enough, the tropomyosin blockade is reestablished, ending the cross bridge activity and contraction. The muscle cell then returns to its resting length.
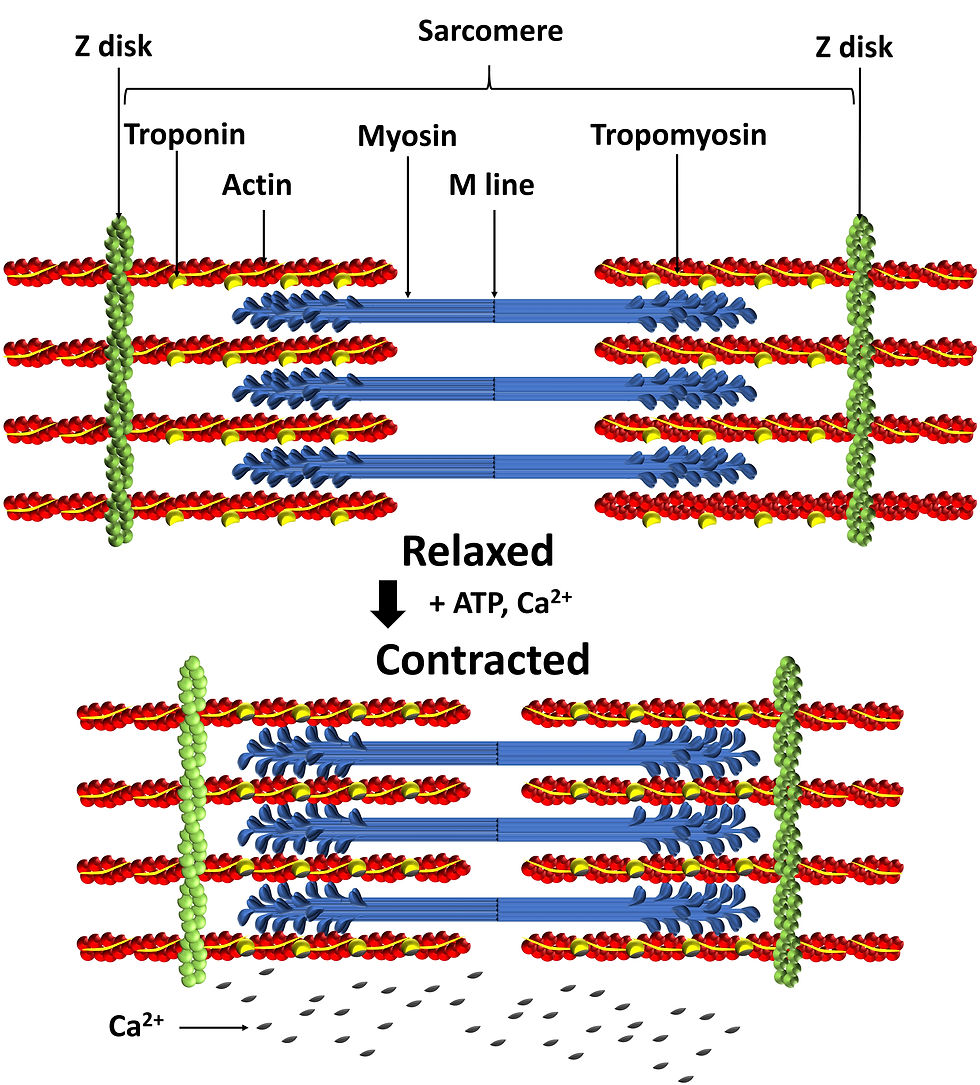
Summary
Muscle cells are long, cylindrical bundles of myofibrils, organelles, and sarcoplasm. Myofibrils make up the bulk of muscle cell volume, accounting for about 80% of the total volume, and are made of repeating units of sarcomeres. The sarcomere is really the contractile unit of skeletal muscle cells, and it is made up of thin filaments (actin), thick filaments (myosin), regulatory proteins (troponin, tropomyosin), and structural proteins (e.g. titin, desmin). Upon stimulation from the nervous system, Calcium binds to troponin, allowing tropomyosin to move out of the way from actin's binding sites. Myosin heads attach to actin's binding sites, forming a cross bridge. Powered with the energy from ATP, the myosin heads pull actin to the center of the sarcomere, shortening the muscle and generating force. Once muscle contraction is no longer needed, intracellular Calcium levels drop. Tropomyosin returns to its original position, blocking the active sites on actin. Myosin heads detach from actin, and the muscle returns to its resting length. Understanding how your skeletal muscles work on a cellular level is very empowering from a health and wellness standpoint. For instance, knowing that Calcium is absolutely essential for muscle contraction, may help you to be more aware of how much Calcium you are getting in your diet. Also, in the case of muscle atrophy (i.e. when muscles shrink in size), it is often due to a breakdown of the proteins that makeup a sarcomere. Conversely, in muscle hypertrophy (i.e. when muscles get bigger in size), there is often an increase in the number of sarcomeres. Finally, it is wonderful to understand how your body is able to move when doing yoga or other forms of movement.
As always, the information presented in this blog post is derived from my own study of neuroscience, human movement, anatomy, and yoga. If you have specific questions about your skeletal muscles, please consult with your physician, physical therapist, or private yoga teacher. If you are interested in private yoga sessions with me, Jackie, you can book services on my website ("Book Online" from the menu at the top of the page), or you can email me at info@lotusyogisbyjackie.com for more information about my services. Also, please subscribe to my website so you can receive my weekly newsletters (scroll to the bottom of the page where you can submit your email address). This will help keep you "in-the-know" about my latest blog releases and other helpful yoga and wellness information. Thanks for reading!
~Namaste, Jackie Allen, M.S., M.Ed., CCC-SLP, RYT-200, RCYT
References:
Clark, M.A. et al. (2018). NASM Essentials of Personal Fitness Training. 6th Edition. Jones & Bartlett Learning. Burlington, MA.
Long, R. (2005). The Key Muscles of Yoga. Scientific Keys, Volume 1. Bandha Yoga Publications.
Marieb, E.N. (2004). Human Anatomy & Physiology – 6th Edition. Pearson Education, Inc. San Francisco, CA.
Neumann, D.A. (2017). Kinesiology of the Musculoskeletal System: Foundations for Rehabilitation. 3rd Edition. Elsevier. St. Louis, MO.
Wisdom, K.M., Delp, S.L., & Kuhl, E. (2015). Use it or lose it: Multiscale skeletal muscle adaptation to mechanical stimuli. Biomechanics and Modeling in Mechanobiology. 14(2): 195 – 215.
Comments