Yoga for Parkinson's Disease Part V - Neurobiology of Brain Areas Affected
- Jackie Allen
- Jun 9, 2023
- 13 min read
Updated: Jun 10, 2023
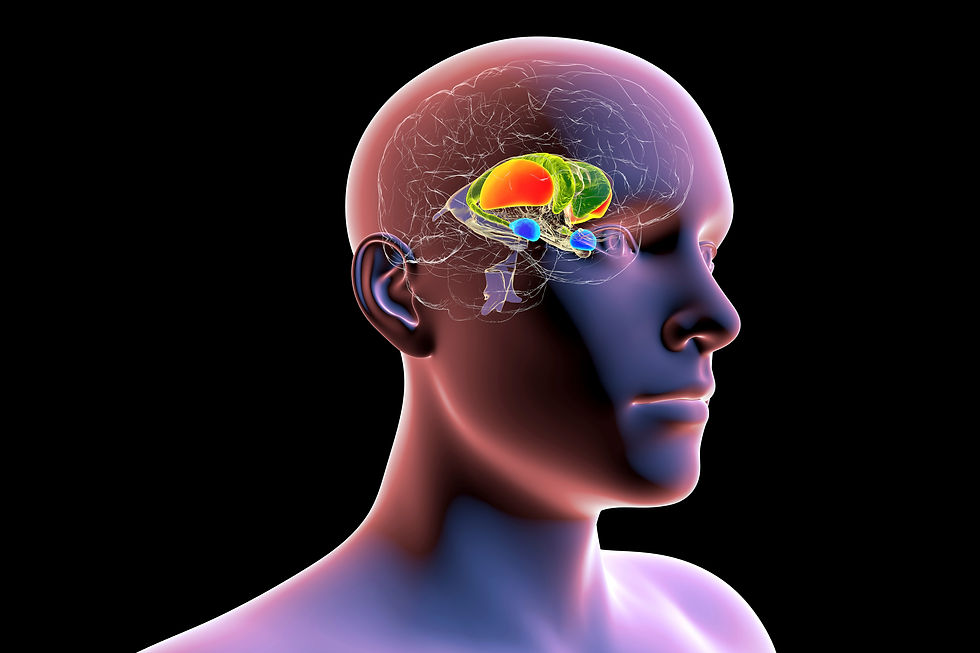
Hey hey readers! Welcome back! In this fifth and final installment of this Yoga for Parkinson’s Disease series, I will review the neurobiology and neurological underpinnings of Parkinson’s Disease (PD). If you have not read the earlier installments in this series, you might want to go back and check those out (part I click here; part II click here; part III click here; and part IV click here).
As reviewed over the course of this series, PD is a progressive neurological disorder, characterized by loss of dopamine-producing neurons in a brain region known as the substantia nigra pars compacta (more on this later in this post). PD results in a myriad of motor and nonmotor symptoms that can be quite debilitating for the person with PD, effectively decreasing quality of life (QOL), independence, and overall functional mobility. As reviewed in part III and IV of this series, yoga and other forms of physical activity (e.g. dancing, running, strength training, etc.) have been shown to be very effective for mitigating the motor and nonmotor symptoms of PD, improving QOL and minimizing the progression of the disease process.
In this current installment, I will provide more in depth information about the neurotransmitter dopamine and the brain areas affected in PD. This post will be heavier on the neuroscience information, but since neuroscience (and dopamine specifically) was the area I studied for my first master’s degree, I am totally geeked up to share this information with you all!
Dopamine
One cannot discuss PD without talking about dopamine (DA) since this is the neurotransmitter that is specifically affected in those with PD. But, to discuss DA, we first need to be on the same page with what a neurotransmitter even is.
What are neurotransmitters? Cells in the human nervous system, known as neurons, communicate with one another by releasing chemical messengers known as neurotransmitters (NTs). A large number of NTs are known, and likely there are more to be discovered. NTs get released from one neuron into a space known as a synapse, which is a small space between neurons. Once the NT crosses the synapse, it binds to receptors on the so-called post-synaptic neuron, where the NT evokes an electrical response in the post-synaptic neuron. This electrical response causes the post-synaptic neuron to do something, and that something depends on what NT is used, the brain region, and the type of post-synaptic cell. Abnormalities in the function of NTs contribute to a wide range of neurological and psychiatric disorders, such as how DA is affected in PD. See the image below for a schematic of what neurons and synapses look like.
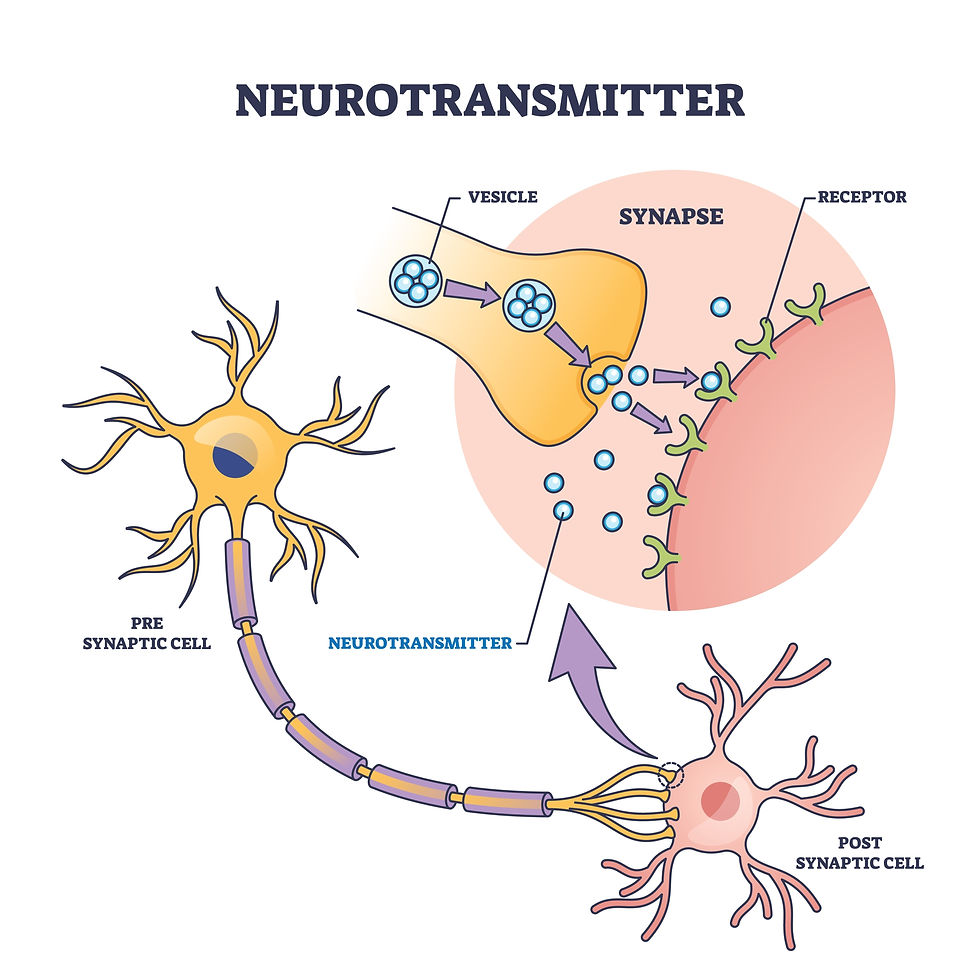
Categories of NTs? There are two main categories of NTs based on size. Neuropeptides are relatively large transmitter molecules composed of 3 to 26 amino acids (AAs). The other group are known as small-molecule NTs, which are much smaller in size than neuropeptides, and include NTs such as glutamate (primary excitatory NT), GABA (primary inhibitory NT), and acetylcholine. Within the small-molecule NT group, there is a subgroup known as the biogenic amines, which includes dopamine (DA), norepinephrine, epinephrine, serotonin, and histamine.
What are biogenic amines? There are five well-established biogenic amine NTs. These five NTs are further subdivided into the catecholamines (which includes DA, norepinephrine, and epinephrine), histamine, and serotonin. The three catecholamines are all derived from a common precursor, which is the amino acid tyrosine. Histamine and serotonin are each individually synthesized by other routes. Biogenic amines are implicated in most psychiatric disorders.
What about dopamine? DA is a hugely important NT in the nervous system. In general, DA is involved in regulating or modulating voluntary movements, motivation, reward, pleasure, learning, and reinforcement. DA is not responsible for directly initiating movement or stimulating skeletal muscles to move; rather, DA plays a modulatory role in movement (more discussed on this later in this post).
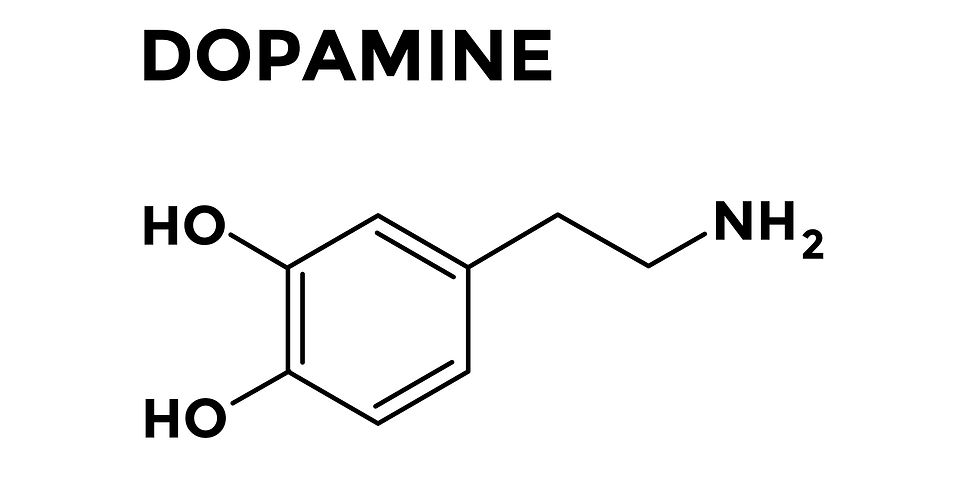
So, now that we have covered the basics about NTs in general and DA specifically, let’s discuss the anatomy of the brain areas involved in PD.
The Basal Ganglia
Tucked into the center-ish of the brain, nestled near the midbrain is a structure known as the corpus striatum, also known as the basal ganglia. This is the primary area of the brain where the PD pathology occurs. The basal ganglia (BG) is generally considered to consist of three main areas – known as the caudate nucleus, putamen, and globus pallidus (aka pallidum). These three areas themselves each consist of masses of neurons, the bulk of which are known as medium spiny neurons (MSNs). In general, these areas help control posture and movement. When discussing the BG, it is super helpful to also consider the following other areas that share some significant connections with the BG – substantia nigra, subthalamic nucleus, thalamus, nucleus accumbens, and amygdala. The image below shows the BG and its parts from a lateral, or side view.
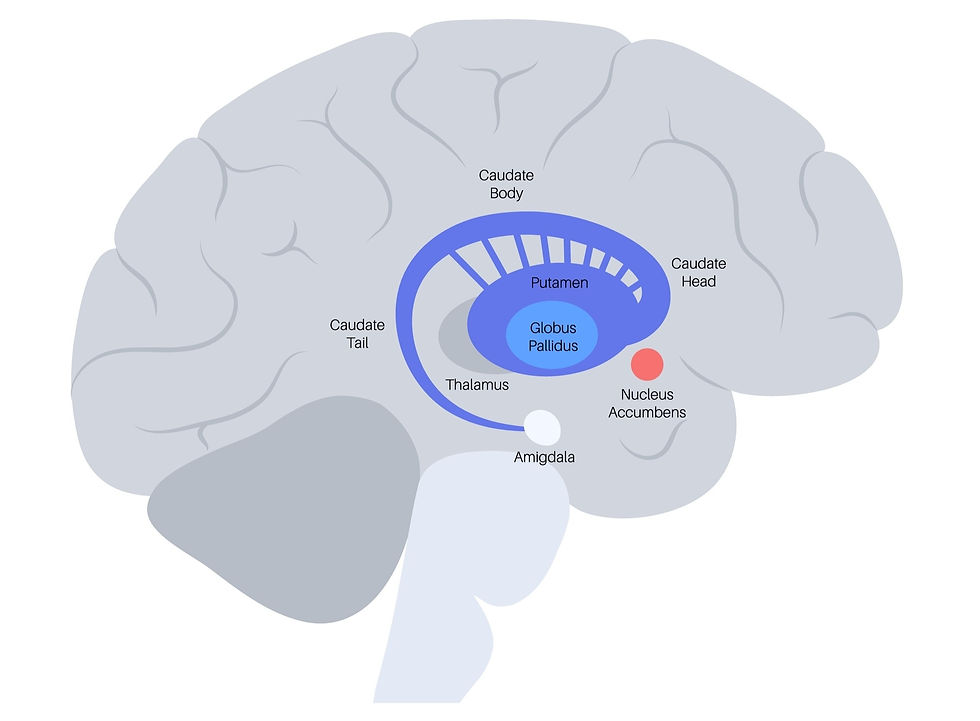
The putamen and globus pallidus (GP) are very close together and seem to form an apparently single structure. Together the putamen and GP are sometimes referred to as the lentiform nucleus. The caudate nucleus and putamen together are sometimes referred to as the neostriatum or more simply the striatum. The MSNs in the striatum use GABA as their NT, meaning that the output of the striatum is inhibitory.
The putamen. The putamen is relatively large and forms the lateral, or more outer, portion of the BG.
The caudate nucleus (CN). The CN consists of a large head and a tapering, curved tail; it is basically c-shaped with a head, body, and tail. The anterior (or front) of the CN is known as the nucleus accumbens (NA), which has connections to the limbic system (the emotional centers of the brain).
The globus pallidus (GP). The GP lies more medial to (inside) the putamen. The GP consists of two divisions known as the external (GPe) and the internal (GPi) segments.
The substantia nigra (SN). The SN is another brain area that is sometimes considered part of the BG. The SN is technically considered part of the midbrain, which is part of the brain stem and right below the BG. The SN has two parts – the pars compacta and the pars reticulata. The substantia nigra pars compacta (SNpc) contains dopaminergic neurons (DAergic), and the substantia nigra pars reticulata (SNpr) actually functions along with the GPi to be the output portion of the BG. This area is not pictured above. But, it is depicted in the image below. As you can see, it lies just south of the BG, right in the midbrain of the brainstem.
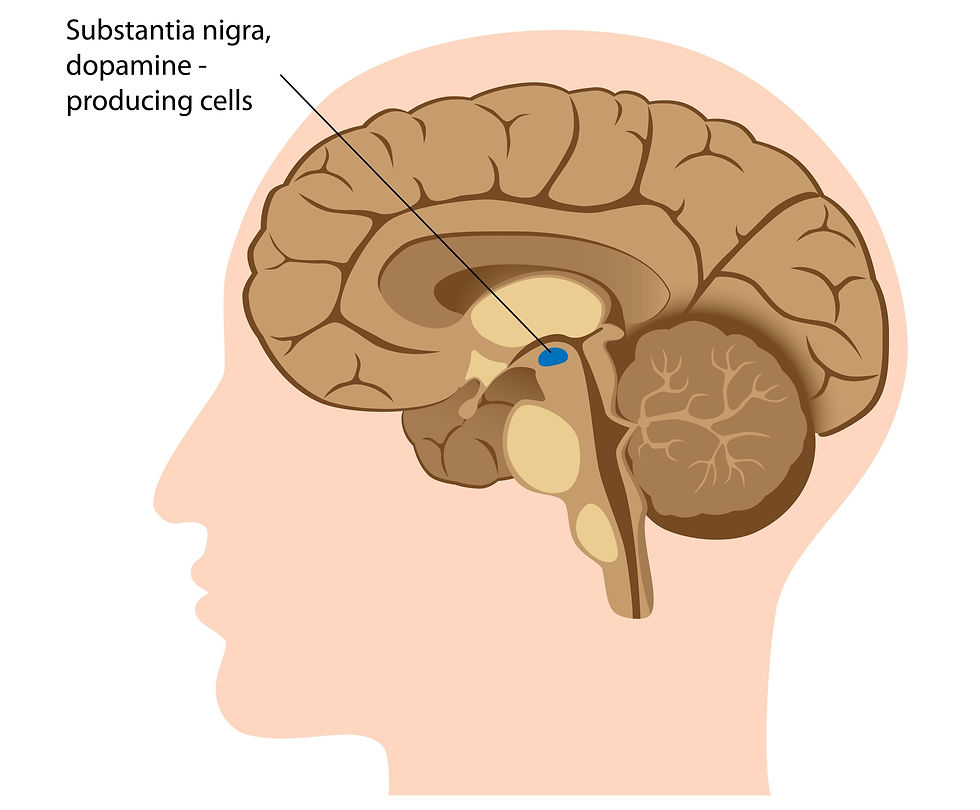
Subthalamic nuclei (STN). The STN is a spindle-shaped structure that is technically part of the diencephalon; is located inferior to (or beneath) the thalamus. It shares many connections between the BG. This area is not pictured above, but it lies just inferior to, or below, the thalamus.
Amygdala. The amygdala is also nearby the BG, but functionally, it is very different; the amygdala is part of the limbic system (the emotional part of the brain), and is especially involved in anger and fear.
Nucleus accumbens (NA). The NA is important in our motivation, reward, and reinforcement pathways, so it is not part of the motor loops that connect to the BG. Rather, the NA is involved in more complex behaviors. This loop can get hijacked in addiction.
Thalamus. The thalamus is essentially a relay station in the brain, where many signals converge for processing and transmission between deeper brain areas and more cortical (or towards to the outer part of the brain) parts of the brain. The BG has many pathways, or loops, to the thalamus.
What Does the BG Do?
Essentially, the BG is responsible for modulating movements, not initiating movement. When a movement is needed by the body, there is first an intention to move, which occurs in the premotor cortex, located on the surface of the brain, towards the front of the head. Then, the movement is initiated via neural impulses from the motor cortex (shown below in the blue area at the top of the brain), which sits just next to the premotor cortex. Neurons in the motor cortex synapse (i.e. make connections) with neurons in the spinal cord after traveling through the brainstem. The neurons in the spinal cord innervate, or connect with, skeletal muscles to cause the actual movement. The image below illustrates this peripheral innervation setup, using the quadricep muscles as an example.
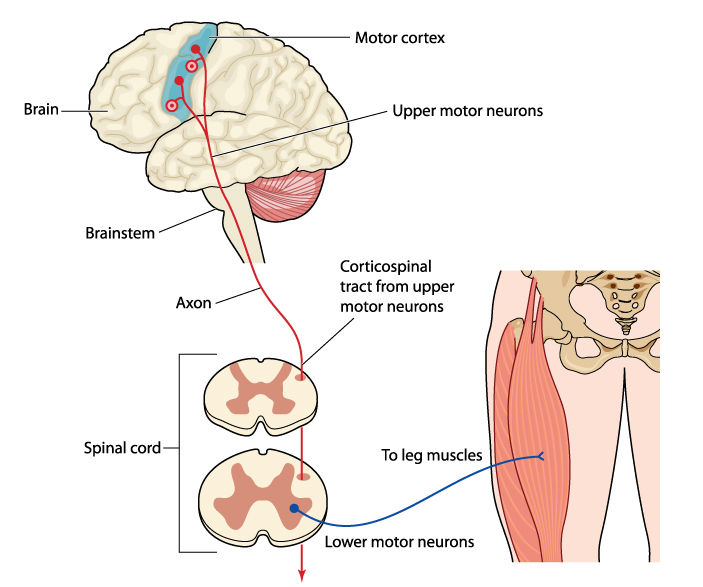
The BG, which lie deep in the cerebral hemispheres, are responsible for modulating the movement while it is happening and helping with switching smoothly between starting and ending a movement. The BG, which relays its neural projections through the thalamus, are required for voluntary movements to be executed and stopped with control, fluency, and precision. The BG also help to inhibit unwanted or inappropriate movements.
The connections from the BG do not go out to the periphery, meaning the muscles in the trunk and limbs. Rather, BG output neurons connect with the frontal cortex (i.e. the part of the brain just behind the forehead). From the frontal cortex, the neurons connect their way into the spinal cord, where the peripheral nerves innervate (i.e. connect with) the individual skeletal muscle itself.
How does this movement modulation occur? When a movement is initiated from the cerebral cortex, impulses discharge to the neuronal pathways that go through the spinal cord out to the periphery. And, impulses also discharge from the cerebral cortex (i.e. surface of the brain) to the BG. The impulses from the cortex to the BG are excitatory and use glutamate as the NT; this excites the medium spiny neurons (MSNs) of the striatum (recall the striatum = caudate nucleus + putamen). The NT used by the MSNs is GABA, which is inhibitory. From here, there are two routes, both of which loop through the thalamus.
First, the so-called “direct pathway,” sends projections to the internal globus pallidus (GPi) and the substantia nigra pars reticulata (SNpr). The net effect of this pathway on the frontal cortex (where the movement is initiated) is excitation from the direct pathway; this supports and facilitates ongoing movements that are occurring. Essentially, the direct pathway sends positive feedback to the cortex, like, “yes, keep doing what you’re doing” or “let’s start this movement.”
The second route is referred to as the “indirect pathway.” In this route, projections from the MSNs in the striatum synapse with neurons in the external globus pallidus (GPe), which then projects to the subthalamic nucleus (STN), and then to the internal GP and the substantia nigra pars reticulata (SNpr) The net effect of the indirect pathway is inhibition of the motor cortex. This has the effect of inhibiting unwanted movements, like, “no, don’t move that far” or “stop this movement now.”
How are the direct and indirect pathways modulated themselves? The direct and indirect pathways can be further modified by the nigrostriatal pathway (this is the pathway affected in PD – more on this in a bit). This pathway goes from the substantia nigra pars compacta (SNpc) to the striatum (i.e. caudate nucleus and putamen), and it uses dopamine (DA) as the NT. Dopaminergic (DAergic) neurons from the SNpc help control and modulate the information flowing through the direct and indirect pathways.
The direct/indirect pathways referred to above are known as the skeletomotor loops in the BG. But, there are other loops that go through the BG circuitry. These include the oculomotor loops (involved in vision), prefrontal loops (involved in thinking skills), and the limbic loop (involved in emotion). The output is basically the same for these other loops, where output neurons project to the thalamus. From the thalamus, these loops project to different regions of the cortex and brain.
So, What Happens in PD?
PD is truly a multisystem brain disorder. While the pathological hallmark of PD is degeneration of DAergic neurons, non-dopaminergic (nonDAergic) pathways can be affected too, such as serotonergic (i.e. NT serotonin) and cholinergic fibers (i.e. NT acetylcholine).
All DAergic neurons in the nervous system are susceptible to various degrees of degeneration, but the primary site of degeneration in PD is the substantia nigra pars compacta (SNpc), which is right in the BG area, next to the substantia nigra pars reticulata (SNpr). These nigrostriatal DAergic neurons are particularly affected, and you can see it on tissue slices as a loss of pigmented cells in that area. When the compacta (SNpc) cells are destroyed, as in PD, the inhibitory outflow of the BG is abnormally high, and thalamic activation of upper motor neurons in the motor cortex is therefore less likely to occur. This can make it so that any movement is difficult to initiate and once initiated, is often difficult to terminate for those with PD. The image below shows a schematic of the different DA pathways found in the human brain. The pathway depicted in blue (nigrostriatal) is the one affected in PD.
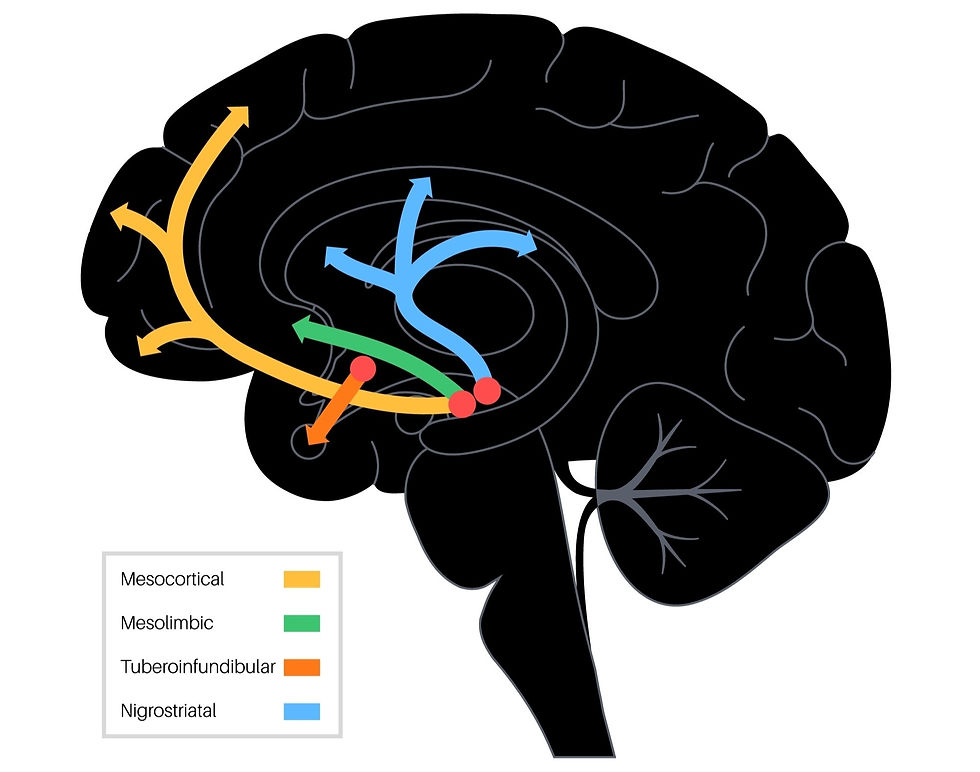
There is a wide range of symptoms seen in those with PD with varying levels of severity. One of the reasons for this variation in symptomology is that there can be differing levels of degeneration across the entire DAergic (and nonDAergic pathways) in the brain. As reviewed in the earlier sections, there are quite a number of neural loops that course through the BG to, and from, other brain areas. So, there are quite a lot of places where dysfunction can occur. Recall, also, that there are motor, emotional, visual, and cognitive loops that run through the BG, so any of these can also be affected by the degeneration of neurons in the SNpc. And also, DAergic systems all over the brain can be affected, contributing to the myriad in symptom presentation.
Summary
Phew! That was quite a lot of information, and I know it was super dense with the neuroscience stuff. Thanks for hanging with me until the end. This blog series has been one of my favorites to research and write about. Not just because of my experience with DA and PD, but also because I am hoping to begin my PhD by August 2025 in the area of PD.
As this entire series has reviewed, PD is a multi-system, progressive neurological disorder in which DAergic neurons in the SNpc degenerate over time. Other NT systems are also affected, including serotonergic and cholinergic pathways, and all DAergic pathways in the nervous system can be affected by the disease. This leads to a wide variation in symptom presentation, where both motor and nonmotor symptoms are common. The symptoms can vary in severity, but typically, without intervention, the disease progresses and symptoms get worse. However, there are interventions that exist and truly help those with PD. Pharmacology (i.e. medications) is commonly prescribed. This often helps with motor symptoms, although there are medications that can sometimes help with nonmotor symptoms. Rehabilitative therapy (e.g. physical therapy, speech therapy, etc.) is also often helpful for those with PD. And, as part III and IV of this series demonstrated, yoga and other forms of physical activity are also helpful for reducing symptoms.
All of the research presented in this series examined participants with mild-to-moderate PD. I was not personally able to find research studies that examined people with severe, or more late-stage PD. Also, I did not come across any studies that looked at people who had just received a PD dx. Furthermore, most of the studies I found examined the effects of yoga/physical activity for about 12 weeks. I did not find any research with long-term follow-up, such as one year or five years out. I would be curious to know how yoga and/or physical activity continue to impact the person with PD over years - for example, does yoga slow progression of the disease?
Please share this information with anyone you think might benefit. Yoga and physical activity can be relatively inexpensive, done at home, and does not require insurance approval. Yoga and physical activity helped reduce nearly all of the PD symptoms, so why not give these various forms of movement a try?
Thank you so much for following this series! I will be taking a little vacay from blog releases for July and August. I will be back in September with some yoga/wellness content. And stay tuned for my next big blog series, all about yoga and physical activity for dementia. This series is still very early on, so I probably wouldn't be releasing any installments for this until maybe end of 2023. But I will keep you all posted!
As always, the information presented in this blog post is derived from my own study of human movement, anatomy and physiology, yoga, and neuroscience. If you have questions about Parkinson's disease specific for you, please follow up with your physician, neurologist, or physical therapist. If you are interested in private yoga and/or personal training sessions with me, Jackie, email me at info@lotusyogisbyjackie.com for more information about my services. Also, please subscribe to my website so you can receive my monthly newsletters (scroll to the bottom of the page where you can submit your email address). This will help keep you "in-the-know" about my latest blog releases and other helpful yoga and wellness information. Thanks for reading!
~Namaste, Jackie Allen, M.S., M.Ed., CCC-SLP, RYT-200, RCYT, NASM-CPT, NASM-CES, NASM-CNC, NASM-SFC, NASM-WLS
References:
Bhalsing, K.S. et al. (2018). Role of Physical Activity in Parkinson's Disease. Annals of Indian Academy of Neurology. 21(4): 242 - 249.
Ban, M. et al. (2021). The Effects of Yoga on Patients with Parkinson's Disease: A Meta-Analysis of Randomized Controlled Trials. Behavioral Neurology. 2021: 1 - 11.
Cheung, C. et al. (2018). Effects of yoga on oxidative stress, motor function, and non-motor symptoms in Parkinson's disease: a pilot randomized controlled trial. Pilot and Feasibility Studies. 4: 162 - 173.
Crossman, A.R. & Neary, D. (2010). Neuroanatomy: An Illustrated Colour Text. Elsevier Limited.
Deuel, L.M. & Seeberger, L.C. (2020). Complementary Therapies in Parkinson Disease: a Review of Acupuncture, Tai Chi, Qi Gong, Yoga, and Cannabis. Neurotherapeutics. 17: 1434 - 1455.
Dong, J., et al. (2016). Current Pharmaceutical Treatments and Alternative Therapies of Parkinson's Disease. Current Neuropharmacology. 14: 339 - 355.
Edinoff, A. et al. (2020). Chronic Pain Treatment Strategies in Parkinson's Disease. Neurology International. 12: 61 - 76.
Elangovan, N. et al. (2020). Hatha yoga improves standing balance but not gait in Parkinson's disease. Sports Medicine and Health Science. 2(2020): 80 - 88.
Fan, B. et al. (2020). What and How Can Physical Activity Prevention Function on Parkinson's Disease? Oxidative Medicine and Cellular Longevity. 1 - 12.
Hao, Z. et al. (2022). Effects of Ten Different Exercise Interventions on Motor Function in Parkinson's Disease Patients - A Network Meta-Analysis of Randomized Controlled Trials. Brain Sciences. 12: 1 - 25.
Iverson, L.L. et al. (2010). Dopamine Handbook. Oxford University Press, Inc. New York, NY.
Jin, X. et al. (2020). The Impact of Mind-Body Exercises on Motor Function, Depressive Symptoms, and Quality of Life in Parkinson's Disease: A Systematic Review and Meta-Analysis. International Journal of Environmental Research and Public Health. 17: 1 - 16.
Khuzema, A. et al. (2020). Effect of home-based Tai Chi, Yoga, or conventional balance exercise on functional balance and mobility among persons with idiopathic Parkinson's disease: An experimental study. Hong Kong Physiotherapy Journal. 40(1): 39 - 49.
Kwok, J.Y.Y. et al. (2019). Effects of Mindfulness Yoga vs Stretching and Resistance Training Exercises on Anxiety and Depression for People with Parkinson Disease- A Randomized Clinical Trial. JAMA Neurology. 76(7): 755 – 763.
Kwok, J.Y.Y., et al. (2017). The effects of yoga versus stretching and resistance training exercises on psychological distress for people with mild-to-moderate Parkinson's disease: study protocol for a randomized control trial. Trials. 18: 1 - 13.
Myers, P.S. et al. (2020). Yoga improves balance and low back pain, but not anxiety, in people with Parkinson's disease. International Journal of Yoga Therapy. 30(1): 41 - 48.
Purves, D. et al. (2004). Neuroscience – 3rd Edition. Sinauer Associates, Inc. Sunderland, MA.
Puymbroeck, M.V. et al. (2018). Functional Improvement in Parkinson's Disease Following a Randomized Trial of Yoga. Evidence-Based Complimentary and Alternative Medicine. 2018: 1 - 8.
Sharma, N.K. et al. (2015). A randomized controlled pilot study of the therapeutic effects of yoga in people with Parkinson's disease. International Journal of Yoga. 8: 74 - 79.
Wu, C. et al. (2021). Effects of Aerobic Exercise and Mind-Body Exercise in Parkinson's Disease: A Mixed Treatment Comparison Analysis. Frontiers in Aging Neuroscience. 13: 1 - 10.
Comments